Authors: Mohammad Rasel, Fahmida Amin, Dlal Gema Hamid Mohammed, March, 2025
1 Description and History
The rapid increase in global energy demand, coupled with growing environmental and economic concerns, has driven a significant shift toward renewable energy sources. Among these, solar photovoltaic (PV) technology has emerged as a key solution due to its ability to harness clean and sustainable energy. Solar PV systems provide a non-polluting energy alternative, contributing to global efforts toward reducing carbon footprints and dependence on fossil fuels. As a result, solar PV has gained widespread adoption and continues to be a focal point in energy research and development.
A solar cell is the fundamental unit of a photovoltaic system, consisting of semiconductor materials that convert sunlight into electrical energy through the photovoltaic effect. These cells are assembled into solar panels or modules, which are typically connected in series or parallel to achieve the required voltage and current levels for various applications1. Solar PV systems are widely used in residential, commercial, and industrial settings, powering everything from small electronic devices to large-scale grid-connected energy systems.
Theoretical principle: The basic physical process of photovoltaic is through which a PV cell converts sunlight into electricity. The Sunlight is composed of various photons packets of solar energy. These photons contain different amounts of energy that correspond to the different wavelengths of the solar spectrum. When photons strike a PV cell, they may be reflected or absorbed, or they may pass right through. The absorbed photons generate electricity2. The efficiency of a solar cell, defined as the percentage of sunlight energy converted into usable electrical energy, varies based on the materials used and the technology employed3.
Solar energy harnessing technologies are primarily categorized into two branches: Concentrated Solar Power (CSP) and Photovoltaic (PV) systems. The concentrated solar power system uses mirrors or lenses which focus extensive sunlight sections to generate heat for driving steam turbines that power electric generators4. PV systems generate electricity directly from sunlight through semiconductor materials in which the photovoltaic effect occurs4.
Both CSP and PV systems can operate independently of conventional power grids or be integrated into existing electrical grids at various transmission or distribution levels, offering flexibility in their applications5.
The Evolution of Solar Photovoltaic (PV) Technology: The development of solar photovoltaic (PV) technology has spanned nearly two centuries, with significant scientific discoveries and engineering breakthroughs shaping its evolution into a mainstream energy source. From the early understanding of the photovoltaic effect to the commercialization of highly efficient solar panels, the journey of solar PV technology is a testament to human innovation and the pursuit of sustainable energy solutions.
Early Discoveries and Inventions: The foundation of solar PV technology dates back to 1839 when French physicist Alexandre-Edmond Becquerel discovered the photovoltaic effect. In his experiment with metal electrodes in an electrolyte solution, he observed that exposure to light generated an electric current6. This discovery laid the groundwork for future advancements in photovoltaic technology.
Further progress was made in 1873, when Willoughby Smith identified the photoconductivity of selenium, revealing that its electrical resistance decreased when exposed to light. Building upon this discovery, Charles Fritts created the first solid-state photovoltaic cell in 1883 by coating selenium with a thin layer of gold. Although this early cell had an efficiency of only about 1%, it demonstrated the feasibility of converting sunlight directly into electricity6.
Theoretical Advancements: The theoretical understanding of photovoltaics took a major leap in 1905 when Albert Einstein published his explanation of the photoelectric effect. His work demonstrated how photons could eject electrons from a material, creating an electric current, a principle that became fundamental to photovoltaic technology. For this groundbreaking contribution, Einstein was awarded the Nobel Prize in Physics in 19216.
Development of Practical Solar Cells: The modern era of solar PV technology began in 1954, when researchers at Bell Laboratories Calvin Fuller, and Gerald Pearson ’s colleague developed a process to dope silicon. This innovation led to the creation of the first p-n junction (diode) by immersing a doped silicon bar on lithium. They reported photovoltaic properties and an astonishing efficiency of 6%, for dopants of boron and arsenic6. Many argue that this event marks the true invention of PV technology because it was the first instance of solar technology that could actually power an electric device for several hours of a day7. This innovation is widely regarded as the birth of modern solar PV technology8.
Space Applications and Early Adoption: The first major application of solar cells was in space technology. In 1958, the Vanguard I satellite became the first spacecraft to use photovoltaic cells as a power source, demonstrating their reliability in extreme conditions9. Following this success, solar technology was integrated into other space missions, increasing global interest in photovoltaic systems.
The 1970s; Energy Crisis and Renewed Interest: The 1973 oil crisis played a critical role in sparking renewed interest in alternative energy sources, including solar power. Governments worldwide increased investments in solar energy research, leading to significant advancements in efficiency and cost reduction. Institutions such as the U.S. Department of Energy along with private sector researchers contributed to the development of more efficient and commercially viable solar panel10.
The 1980s; Thin-Film Technology and Efficiency Gains: The 1980s saw significant improvements in thin-film solar cell technology, which used less material and had the potential for lower manufacturing costs. In 1980, the Institute of Energy Conversion at the University of Delaware developed the first thin-film solar cell11 . These advancements made solar technology more accessible to a broader market.
The 1990s–2000s; Commercialization and Expansion: During the 1990s and early 2000s, solar PV technology experienced rapid commercialization. Government incentives, environmental awareness, and technological improvements led to widespread adoption. In this period the advancements in solar technology led to significant improvements in efficiency. By the early 1990s, solar panel efficiencies had reached around 15-20% for commercially available panels.The early 2000s saw significant technological breakthroughs in solar energy. Innovations in thin-film solar cells, multi-junction cells, and concentrated photovoltaic systems pushed efficiencies beyond 20%. The introduction of large-scale manufacturing processes, particularly in China, led to economies of scale that drastically reduced costs12.
The 2010s; Global Growth and Cost Reduction: The 2010s marked a period of rapid expansion for solar photovoltaic (PV) technology, characterized by significant cost reductions and increased global adoption. Manufacturing costs for solar modules dropped by approximately 90% during this decade, driven by technological advancements and economies of scale13. These substantial decreases made solar power increasingly competitive with conventional energy sources. Countries such as China, Germany, and the United States invested heavily in large-scale solar farms, integrating solar energy into their national power grids. China, for instance, became the world’s largest producer of photovoltaic power by 2015, surpassing Germany, and continued to lead in solar installations throughout the decade14. Germany maintained its leadership in solar installations, contributing significantly to its renewable energy portfolio15.The United States also saw substantial growth in utility-scale solar projects, with installations increasing from 2.6 GW in 2010 to over 35 GW by 202016.
Present Day; A Mainstream Energy Source: As of 2025, solar photovoltaic (PV) technology has become a mainstream energy source, contributing significantly to global electricity generation. Continuous advancements in materials science, energy storage, and grid integration have enhanced the efficiency and reliability of solar power. For instance, recent developments in photovoltaic materials have led to higher efficiency rates, making solar energy more competitive with traditional energy sources17.
In terms of global renewable energy targets, numerous countries have set ambitious goals to increase their renewable energy capacity. The International Renewable Energy Agency (IRENA) outlines various pathways and strategies to accelerate the deployment of solar PV to meet these objectives18.These developments underscore the pivotal role of solar PV in the transition toward a sustainable energy future, ensuring that solar energy remains a key driver in the global shift towards renewables. According to National Renewable Energy Laboratory (NREL) the projected cumulative global PV capacity has been illustrated in Figure 1.
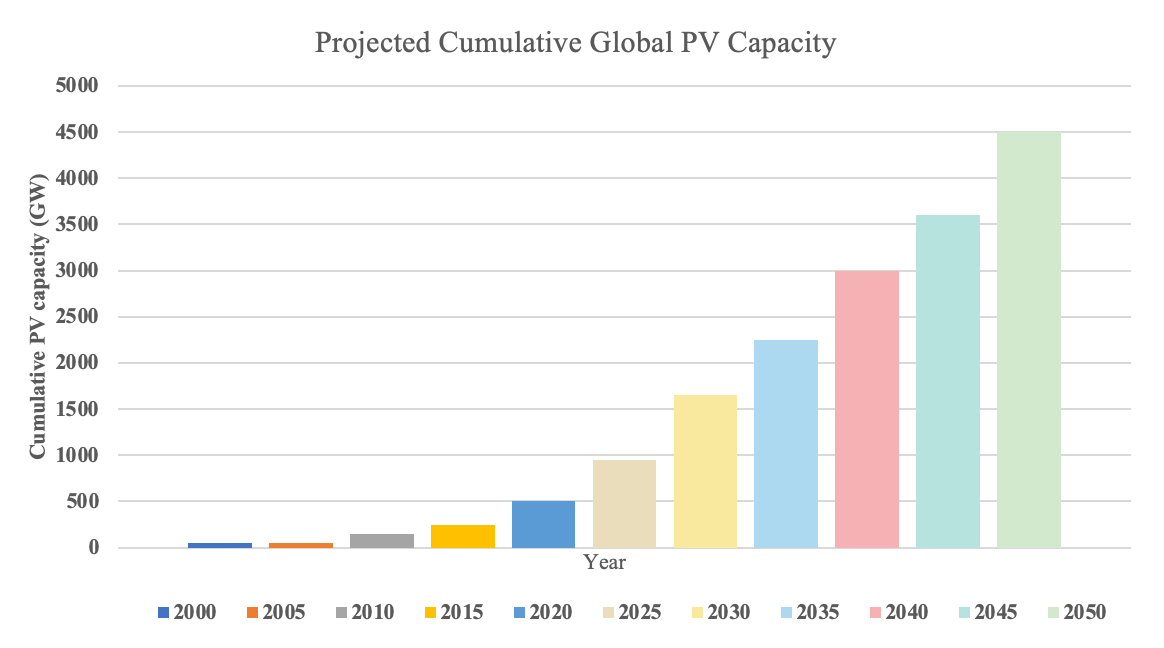
2 Economic Performance
In the last two decades, solar photovoltaic (PV) technology has undergone significant advancement. Which results to a dramatic reduction in costs and making it an increasingly competitive alternative to traditional energy sources. Initially, in the 1970s, solar PV technology was prohibitively expensive, with costs around $76 per watt, primarily due to inefficiencies and the small scale of production20. However, solar PV has become one of the most affordable sources of energy today due to a combination of technological innovations, improved manufacturing processes, and economies of scale.
Technological Advancements and Cost Reductions: One of the major drivers behind the decline in solar PV costs has been technological progress. Over the years, improvements in manufacturing techniques, materials, and design of solar cells have led to higher efficiency and lower production costs. Since 2010, the global average cost of solar PV has decreased by over 80%, driven by innovations in materials, manufacturing processes, and system designs. These advancements, such as the development of more efficient photovoltaic cells like PERC, and improvements in inverters and energy storage, have made solar PV more efficient and reliable, thus reducing installation costs and increasing energy output 21. As the industry has scaled, economies of scale have further reduced costs, making solar PV more competitive with traditional energy sources, with the cost of utility-scale solar dropping from over $350 per kilowatt in 2010 to $50–$60 per kilowatt by 202022.
Economies of Scale and Market Competition: Another important factor contributing to cost reductions in solar PV is economies of scale. As the demand for solar technology has increased, manufacturers have been able to produce solar cells in larger quantities, thus reducing unit costs. According to a report by the International Renewable Energy Agency (IRENA), the global cost of solar PV has fallen by more than 80% since 2010, primarily due to increased production volumes and technological improvements22. This reduction in cost has made solar PV more accessible, allowing it to compete with conventional energy sources, such as fossil fuels, in terms of both affordability and efficiency. According to the National Renewable Energy Laboratory (NREL), economies of scale and increased competition have significantly lowered the costs of utility-scale solar installations, making them more economically viable for large-scale energy generation23. For example, the cost of utility-scale solar projects has dropped from over $350 per kilowatt in 2010 to around $50–$60 per kilowatt by 2020, largely due to higher production volumes and technological innovations. This ongoing competitive pressure is expected to continue fostering advancements in solar technology, driving down costs even further and enhancing the economic performance of solar PV systems22.
Levelized Cost of Electricity (LCOE):

The Levelized Cost of Electricity (LCOE) is a critical metric used to evaluate the cost-effectiveness of different energy generation technologies, including solar PV. It represents the per-unit cost of electricity generated by a specific technology over its lifetime, accounting for all costs of installation, operation, and maintenance. According to the International Renewable Energy Agency (IRENA), the LCOE of solar PV has dropped significantly in recent years due to technological advancements and cost reductions in installation and maintenance. In 2020, the global average LCOE for utility-scale solar PV ranged from $20 to $60 per megawatt-hour (MWh), making it one of the most affordable energy sources22. This sharp decline in LCOE is primarily attributed to economies of scale, improved efficiencies in solar panel technology, and the increased availability of financing options. As the solar industry continues to evolve, it is expected that the LCOE for solar PV will continue to fall, further enhancing its competitiveness against traditional fossil fuels23.
Development of the Solar PV Industry in Terms of Revenue: The solar photovoltaic (PV) industry has undergone substantial growth, evolving into a multi-billion-dollar global sector driven by both technological advancements and increasing demand for renewable energy. In 2020, the global revenue of the solar industry surpassed $100 billion, with projections indicating that it will continue to grow, reaching approximately $300 billion by 203024. The expansion of the solar PV market is largely fueled by increased deployment, technological innovations, and government incentives aimed at reducing carbon emissions and transitioning to cleaner energy sources.
According to BloombergNEF, China consistently produces around 70% of the world’s solar panels, which has made it the dominant player in the global solar manufacturing market24.The revenue generated from China’s solar industry alone was estimated at over $50 billion in 202025. Several large Chinese companies, including LONGi Green Energy and JinkoSolar, have become global leaders in solar panel production, contributing significantly to the industry’s financial growth. Similarly, countries like the United States and Germany have also witnessed substantial increases in the production of solar components, contributing to the global market’s expansion.
Technological advancements in solar PV have played a pivotal role in lowering production costs, improving panel efficiency, and enhancing energy storage capabilities. These improvements have helped reduce the levelized cost of electricity (LCOE) for solar PV, making it one of the most affordable forms of energy generation. As of 2020, the LCOE for utility-scale solar PV ranged from $20 to $60 per megawatt-hour (MWh), which is comparable to or even lower than conventional fossil fuels22. The industry’s revenue is also bolstered by the growth in global installations, as more countries and regions adopt solar as a primary energy source, leading to increased demand for solar panels, inverters, and energy storage systems.
Moreover, the development of large-scale solar farms and the increase in investments from both private and public sectors have been critical drivers of the market’s revenue growth. In 2020 alone, over 120 gigawatts (GW) of solar capacity were installed globally, with countries like China, the United States, and India accounting for the majority of new installations26. This growing demand for solar technology has attracted substantial capital investment, further contributing to the expansion of the solar PV market.

The combination of falling costs, advancements in technology, and increased installation capacity has made solar PV a key contributor to the global energy transition which can be seen in Figure 3. As the market matures, solar PV companies are expected to continue driving innovation, improving efficiency, and expanding their reach, further enhancing their revenue potential and reinforcing the industry’s position as a leader in the renewable energy sector.
3 Ecological Performance
Solar photovoltaic (PV) technology is a prominent renewable energy source, recognized for its minimal environmental impact during operation. Unlike fossil fuel-based energy generation, solar PV systems do not produce air pollution or greenhouse gases while generating electricity27.
Ecological Impacts: The establishment of large-scale solar PV facilities can lead to habitat loss, soil erosion, and alterations in local hydrology. These environmental changes may adversely affect native flora and fauna, particularly in ecologically sensitive areas. The Union of Concerned Scientists highlights that the environmental impacts of solar power, including land use and habitat loss, can vary significantly depending on the technology and scale of the system28. To mitigate this, it required careful planning and management. Implementing strategies such as agrivoltaics, pollinator-friendly designs, and the utilization of degraded lands can mitigate negative impacts and promote biodiversity. Engaging local communities in decision-making processes is crucial to harmonize renewable energy development with ecological preservation and social acceptance.
Comparative Analysis with Alternative Energy Technologies: When evaluating the ecological impacts of solar PV relative to other energy technologies, several factors emerge:
- Air Pollution: Solar PV systems do not emit air pollutants during operation, unlike fossil fuel-based power plants, which release sulfur dioxide (SO₂), nitrogen oxides (NOₓ), and particulate matter, contributing to air quality degradation and health issues27.
- Land Use Efficiency: While nuclear and natural gas power plants have higher power densities (240 and 480 W/m², respectively), solar PV installations average about 7 W/m². However, integrating PV systems into existing structures, such as rooftops, can enhance land-use efficiency29.
- Resource Availability and Sustainability: Solar energy is abundant and renewable, whereas fossil fuels are finite and associated with extraction-related environmental disturbances. Wind and hydroelectric power also offer renewable alternatives with varying ecological footprints29.
Reduction in Greenhouse Gas Emissions:

In the last few decades, the lifecycle greenhouse gas (GHG) emissions associated with solar photovoltaic (PV) systems have significantly decreased due to advancements in technology, manufacturing processes, and efficiency improvements. Early assessments of PV systems, particularly from the late 1990s and early 2000s, reported relatively higher GHG emissions. A study by Jungbluth (2000) estimated lifecycle GHG emissions for various PV systems to be in the range of 39–110 grams of CO₂-equivalent per kilowatt-hour (g CO₂-eq/kWh), with energy payback times (EPBT) of 3–6 years31. Since then, increased module efficiency, optimized manufacturing processes, and material innovations have contributed to a significant reduction in emissions.
Recent assessments reflect these technological advancements. A 2012 fact sheet from the National Renewable Energy Laboratory (NREL) reported that harmonized lifecycle GHG emissions for c-Si PV systems were significantly lower than earlier estimates32. A more recent 2023 NREL report further highlighted that modern utility-scale PV systems exhibit lifecycle GHG emissions of approximately 40 g CO₂-eq/kWh, showcasing continued improvements in PV technology and manufacturing efficiency32. When compared to conventional fossil fuel-based electricity generation, the reduction in GHG emissions from PV systems is even more pronounced. Coal-fired power plants, for example, have lifecycle GHG emissions approximately 20 times higher than those of solar PV systems32. The significant decline in lifecycle GHG emissions from solar PV systems underscores the positive environmental impact of technological advancements in the solar industry. As PV technologies continue to evolve, further reductions in GHG emissions are anticipated, reinforcing the role of solar energy in mitigating climate change. The scenario of greenhouse gas emission for different energy sources has been illustrated in Figure 4.
Decreased Energy Payback Time: The energy payback time (EPBT) of solar photovoltaic (PV) systems has markedly improved, reflecting significant advancements in technology and manufacturing processes. EPBT measures the duration required for a PV system to generate the amount of energy expended in its production, serving as a critical indicator of the system’s sustainability. Contemporary PV systems now exhibit EPBTs of less than 2 years, highlighting the substantial progress made in enhancing the sustainability and energy efficiency of solar energy technologies33.
Recent studies indicate a further reduction in EPBTs. A 2023 life cycle assessment by the National Renewable Energy Laboratory (NREL) reported that modern utility-scale PV systems in the United States exhibit EPBTs between 0.5 and 1.2 years, depending on the system’s location and local solar radiation levels33. A shorter EPBT allows a PV system to contribute clean energy more quickly. For instance, a system with an EPBT of one year starts generating net-positive energy for the remaining 24–29 years of its lifespan, reducing fossil fuel dependency and minimizing ecological damage.
Resource Optimization and Material Usage: Advancements in PV technology have also led to more efficient use of materials. The transition from early, less efficient panels to modern designs has reduced the amount of semiconductor material required per unit of energy produced. For example, the development of perovskite-silicon tandem cells has demonstrated efficiencies exceeding 25%, indicating a promising path toward higher performance with potentially lower material inputs34.Moreover, ongoing research aims to enhance the recyclability of PV materials, addressing environmental concerns associated with panel disposal. Studies are exploring the recovery of valuable materials like silicon, silver, and rare earth elements from end-of-life panels, promoting a circular economy and reducing the need for new raw materials.
These continuous improvements in material efficiency and resource optimization have significantly advanced PV technology, leading to higher energy conversion rates and more sustainable manufacturing practices. These developments not only enhance the economic feasibility of solar energy but also contribute to environmental conservation through reduced material usage and improved recyclability.
Enhanced Recycling and End-of-Life Management: As the deployment of PV systems has expanded, so has the emphasis on sustainable end-of-life management. Initially, in the 1970s and 1980s, recycling efforts were rudimentary, focusing mainly on recovering aluminum frames and glass from decommissioned panels. As PV technology matured, so did recycling processes, incorporating methods to reclaim valuable materials such as silicon, silver, and rare earth elements35.
Recent developments have further enhanced recycling efficiency. For instance, Pan Pacific Recycling in Brisbane has pioneered techniques to extract silver and copper from old solar panels without generating toxic emissions or contributing to landfill waste. This initiative addresses the growing concern of solar waste, especially as demand for secondhand panels declines due to the influx of affordable new panels from manufacturers like China36.
Looking ahead, the International Renewable Energy Agency (IRENA) estimates that by 2050, recycling or repurposing PV panels could unlock up to 78 million tonnes of raw materials globally, valued at over $15 billion. This underscores the immense potential of EoL management in contributing to a circular economy and reducing the environmental footprint of solar energy37.
Mitigation Strategies: To address the ecological challenges of solar PV deployment, several strategies are being implemented. Agrivoltaics involves co-locating solar panels with agricultural activities, enabling both crop production and energy generation38. Pollinator-friendly solar initiatives integrate native vegetation into solar installations, enhancing biodiversity and supporting local ecosystems39. Additionally, utilizing degraded lands, such as brownfields or abandoned mining areas, for solar development helps minimize impact on pristine environments while benefiting local communities40. These strategies aim to make solar energy development more sustainable and environmentally beneficial.
4 Social Impact
The social acceptance of solar photovoltaic (PV) technology has undergone a significant transformation over the past few decades, evolving from a niche interest to a widely embraced energy solution. This shift is evident through various studies, statistics, and literature that highlight the growing public support and adoption of solar PV systems globally.
Early Perceptions and Adoption: In the initial stages, solar PV technology faced skepticism and limited adoption due to high costs, technological uncertainties, and a lack of awareness. For instance, a study conducted in Malaysia in 2011 revealed that the public was hesitant to invest in photovoltaics, primarily because they did not fully understand the incentives and significant socioeconomic benefits associated with the technology41.
Influence of Social Factors: Research indicates that social dynamics play a crucial role in the adoption of solar PV technology. A study by the London School of Economics found that households, businesses, and farms are more likely to install solar panels if others in their neighborhood have already done so, especially when existing installations are highly visible42. This phenomenon, known as social contagion, suggests that visible adoption within a community can alleviate concerns about investment risks and encourage others to follow suit.
Statistical Growth and Public Support: The increasing social acceptance of solar PV is reflected in its rapid adoption rates. In Germany, for example, the share of renewable energy in electricity production rose from 3.5% in 1990 to 52.4% in 2023, with solar power contributing significantly to this growth43. Public opinion surveys further underscore this acceptance; a 2014 survey showed that 94% of Germans supported the expansion of renewable energies, with more than two-thirds open to having renewable power plants close to their homes. Similarly, in California, solar power has seen substantial growth, with the state achieving a total of 46,874 MW of installed solar capacity by the end of 2023, enough to power 13.9 million homes. This expansion is attributed to high public support, favorable policies, and declining solar costs44.
Recent Trends and Innovations: Innovative applications of solar PV technology have further enhanced its social acceptance. The concept of “balcony solar” has gained popularity in urban areas, particularly in Germany, where approximately 1.5 million households have installed plug-in solar panels on their balconies. These systems offer a cost-effective and accessible means for apartment dwellers to participate in renewable energy generation, reflecting a societal shift towards embracing decentralized energy solutions45.
Challenges and Barriers: Despite the positive trajectory, challenges persist. Barriers such as financial constraints, regulatory hurdles, and informational deficits can effect this adoption. A meta-analysis highlighted that perceived benefits, environmental concern, and social norms significantly influence individuals’ intentions to adopt residential PV systems46. Addressing these factors through targeted policies and community engagement is essential to further enhance social acceptance.
Job Creation and Economic Opportunities:
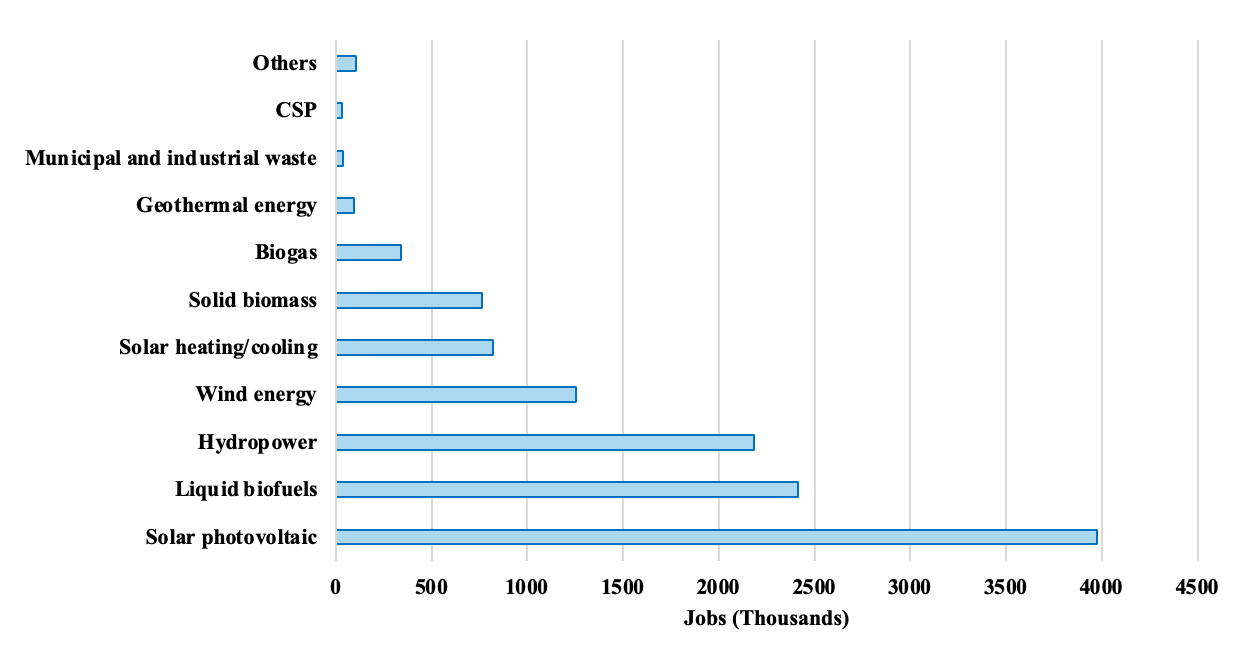
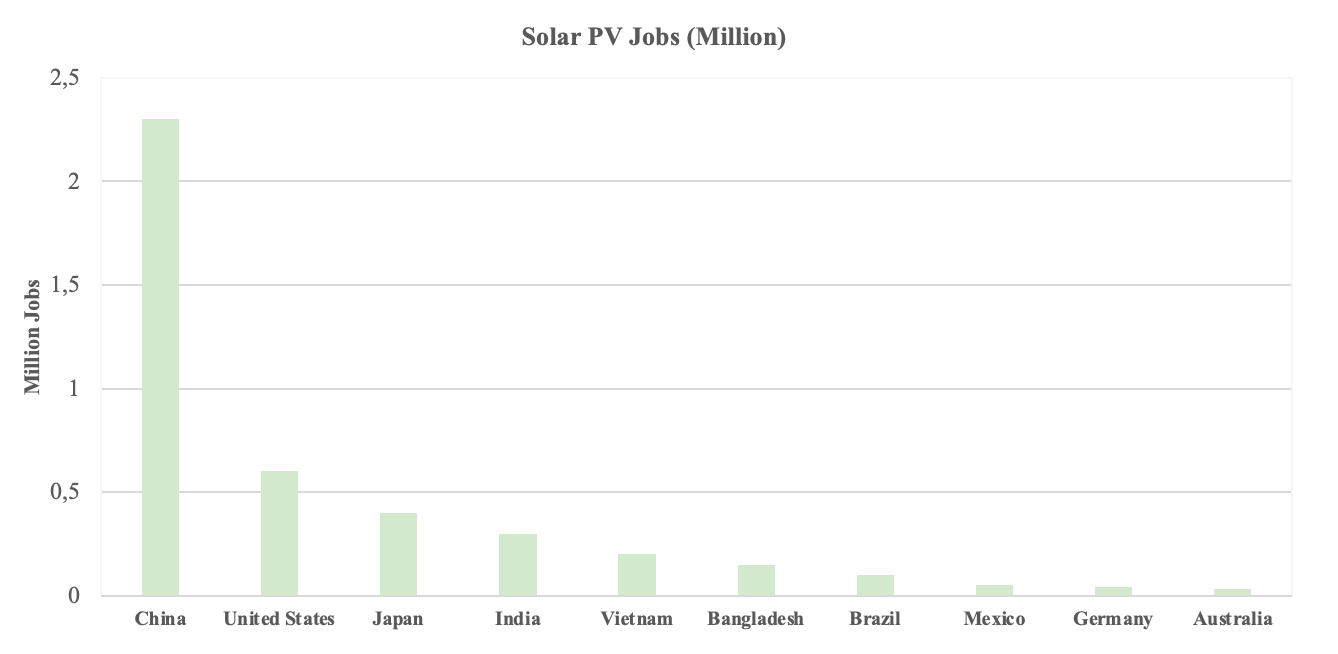
The adoption of solar photovoltaic (PV) technology has created significant job opportunities across sectors like manufacturing, installation, maintenance, and research & development (R&D) as shown in Figure 5 and Figure 6. According to the International Renewable Energy Agency (IRENA), the solar PV sector alone employed approximately 4 million people globally in 2021, accounting for the highest share among all renewable energy sources47. These jobs span multiple skill levels, from low-skilled panel installation to high-tech engineering and R&D roles, ensuring employment opportunities for a broad segment of the population.
Furthermore, the solar energy industry provides localized employment, particularly in rural and underdeveloped areas where grid connectivity remains a challenge. The decentralized nature of solar PV systems allows small-scale enterprises and cooperatives to thrive, enabling individuals to generate their own electricity and even participate in microgrid systems, fostering economic independence. For instance, in Sub-Saharan Africa and South Asia, small and medium-sized enterprises (SMEs) involved in solar panel sales, installation, and maintenance have seen a surge in demand, thus contributing to job creation and economic empowerment in marginalized regions48.
Moreover, investment in solar PV technology supports a just transition from fossil fuel-based industries to a cleaner and more sustainable energy system. In countries where traditional fossil fuel industries have dominated, reskilling programs aimed at transitioning workers into the solar sector have played a crucial role in mitigating job displacement. As a result, solar energy contributes not only to environmental sustainability but also to social stability by ensuring a smooth employment transition49.
Health Benefits and Avoided Mortality: Solar PV technology directly contributes to improved public health by reducing reliance on fossil fuel-based power generation, which is a major source of air pollution and greenhouse gas emissions. The burning of coal, oil, and natural gas releases pollutants such as particulate matter (PM2.5), nitrogen oxides (NOx), and sulfur dioxide (SO2), which are known to cause respiratory diseases, cardiovascular conditions, and premature mortality 50. According to a study by the Health Effects Institute (2020), air pollution from fossil fuel combustion was responsible for an estimated 8.7 million premature deaths globally in 201851. By replacing fossil fuels with solar PV, these adverse health effects can be substantially reduced, leading to lower disease burden and improved life expectancy.
In addition to reducing outdoor air pollution, solar PV technology also minimizes health hazards associated with indoor air pollution. In many developing countries, households without access to electricity rely on kerosene lamps, biomass, or diesel generators for lighting and cooking. These sources emit harmful pollutants that contribute to respiratory illnesses, particularly among women and children who spend more time indoors52.
Furthermore, solar PV technology contributes to improved healthcare services by providing reliable electricity to medical facilities, especially in remote regions where grid access is unreliable. Studies indicate that electrified health centers experience improved patient care, better storage of vaccines and medicines (due to refrigeration), and increased medical equipment functionality, ultimately reducing maternal and child mortality rates. In rural clinics of Sub-Saharan Africa, for example, solar-powered refrigeration has played a pivotal role in vaccine distribution, significantly reducing preventable diseases53.
Reduction of Social Inequality: Access to affordable and sustainable energy is a key factor in reducing social and economic disparities. Solar PV technology has been instrumental in bridging the energy access gap, particularly in underserved and remote regions. According to the International Energy Agency (IEA), over 700 million people worldwide still lack access to electricity, with the majority residing in Sub-Saharan Africa and South Asia54.
Solar PV-powered microgrids and off-grid systems enable equitable energy distribution, ensuring that even the poorest communities can benefit from reliable electricity. This access to energy improves educational outcomes by providing lighting for evening study, powering digital learning tools, and ensuring schools can function effectively55. Additionally, women and children, who often bear the brunt of energy poverty, experience significant social benefits through solar PV deployment. Electrification reduces time spent on labor-intensive household chores, such as collecting firewood or manually grinding grains, thereby allowing more opportunities for education and income-generating activities.
By reducing energy poverty and enabling economic participation, solar PV technology fosters greater social inclusion and mobility. In many regions, electrification through solar energy has contributed to gender empowerment by supporting women-led solar enterprises and increasing female participation in clean energy initiatives. Programs such as the Barefoot College’s solar engineering training for rural women in India and Africa highlight the role of solar technology in promoting gender equality and sustainable development56.
Negative Social Impacts: While solar photovoltaic (PV) systems are widely recognized for their environmental benefits, they can also pose certain social challenges in some extend. The construction phase of large-scale solar farms generates noise, which may disrupt nearby communities, though operational PV systems are generally quiet57. Health concerns also arise from the presence of hazardous materials like lead or cadmium in some PV panels, which, if damaged or improperly disposed of, could contribute to environmental contamination28. Additionally, large-scale solar farms require significant land, potentially leading to habitat disruption, biodiversity loss, and conflicts with existing land uses such as agriculture or residential28. However, these impacts can often be mitigated through sustainable planning, community engagement, and adherence to environmental regulations.
5 Political and Legal Aspects
The global advancement of solar photovoltaic (PV) technology have been profoundly influenced by the divers of national policies. These policies, encompassing financial incentives, regulatory frameworks, and strategic initiatives, have been pivotal in shaping the solar energy landscape. The detailed examination of the policies adopted by various countries has been illustrated below.
- Germany
Germany’s solar photovoltaic (PV) sector has been profoundly influenced by a series of policies aimed at promoting renewable energy adoption and integration. These policies have evolved over time, reflecting the dynamic nature of the energy market and technological advancements. Few key policies are illustrated below:
Renewable Energy Sources Act (Eeg) 2014: The 2014 amendment of the Renewable Energy Sources Act marked a significant shift in Germany’s approach to renewable energy incentives. This version introduced a transition from fixed feed-in tariffs to an auction-based system for determining remuneration rates for renewable energy sources, including solar PV. The objective was to enhance market integration and cost efficiency in renewable energy expansion. Specific deployment corridors were established to control the growth of various technologies, ensuring a balanced and sustainable increase in renewable energy capacity58.
Feed-In Tariffs Adjustments: Germany’s feed-in tariff (FiT) system has undergone multiple adjustments to align with market conditions and technological progress. As of July 2014, the FiTs ranged from 3.33 €/kWh for hydropower facilities over 50 MW to 12.88 €/kWh for solar installations on buildings up to 30 kWp. These tariffs were designed to provide long-term security to renewable energy producers, with rates reflecting the cost of generation for each technology. The regular degression of tariffs aimed to encourage innovation and cost reductions within the industry59.
Market Integration Measures: In November 2024, the German cabinet approved a plan requiring new wind and solar power plants to sell electricity independently on the open market, moving away from guaranteed prices. This policy targets facilities as small as 25 kilowatts, aiming to better integrate renewable energy into Germany’s energy system and manage electricity surpluses that can lead to negative prices. The reform encourages operators to market their power directly and invest in storage solutions, promoting a more resilient and market-responsive renewable energy sector60.
Zero VAT Rate for Solar Installations (2024): To incentivize the adoption of solar energy, the German government proposed a zero Value Added Tax (VAT) rate for the supply and installation of solar modules, effective from January 2023. This policy eliminates VAT charges for operators of private PV systems on both the purchase and installation, reducing the overall cost and encouraging more homeowners and businesses to invest in solar energy solutions61.
Moreover, Germany’s solar industry has experienced significant growth, with solar power accounting for an estimated 14% of net public electricity generation in 2024, up from less than 0.1% in 2000. This expansion has been supported by policies such as the Renewable Energy Sources Act (EEG) and various financial incentives. These policies and measures reflect Germany’s commitment to fostering the development and diffusion of solar photovoltaic (PV) technology, while continually adapting to market developments and technological advancements62.
- China
China has ascended as a global leader in solar energy deployment, driven by strategic government policies and economic planning. China’s rapid development and diffusion of solar photovoltaic (PV) technology have been significantly influenced by a series of strategic policies and initiatives implemented over the past few decades, among them the key policies are highlighted below:
Early Initiatives and Industrial Policies: The foundation for China’s solar PV industry was laid during the Sixth Five-Year Plan (1981–1985), which first addressed government support for solar PV panel manufacturing. Subsequent plans continued this emphasis, fostering growth in both manufacturing and technological innovation. In the late 1990s and early 2000s, programs like the Brightness and Township Electrification initiatives aimed to provide electricity to approximately 23 million people in remote areas, significantly contributing to the early development of the PV industry63.
Stimulus Measures and Feed-in Tariffs: The global financial crisis of 2007–2008 prompted China to invigorate its then-struggling solar industry through significant stimulus efforts. In 2009, the government launched the “Golden Sun” program, offering financial subsidies, technological support, and market incentives to promote solar power development64. This initiative aimed to reduce dependence on foreign markets and mitigate trade tensions with the European Union and the United States. In 2011, China introduced nationwide feed-in tariffs (FITs) to guarantee favorable electricity prices for solar power producers, further accelerating domestic installations65.
Recent Developments and Policy Adjustments: China added 357 gigawatts (GW) of combined solar and wind power capacity in 2024, representing a 45% increase in solar capacity and an 18% increase in wind capacity compared to the end of 202366. This significant growth enabled China to surpass its 2030 renewable energy targets six years ahead of schedule66.
In response to this rapid expansion, in February 2025, China’s National Development and Reform Commission (NDRC) announced plans to scale back subsidies for new renewable energy projects. The new policy requires projects approved after June 2025 to participate in market-based bidding for electricity payments, reflecting the maturation of the industry and aiming to ensure its sustainable development67.
These policies collectively highlight China’s strategic approach to fostering the growth of its solar PV industry, balancing rapid expansion with sustainable practices and market-oriented reforms.
- India
India’s advancement and widespread adoption of solar photovoltaic (PV) technology have been significantly influenced by a series of strategic policies and initiatives implemented over the past decade. The cornerstone of these efforts is the National Solar Mission, inaugurated in 2010 with an initial target of 20 gigawatts (GW) of solar capacity by 2022. Recognizing the potential of solar energy, this target was ambitiously revised in 2015 to 100 GW68. This mission has been instrumental in accelerating solar power development across the country.
To further bolster solar energy adoption, the Pradhan Mantri Kisan Urja Suraksha evam Utthaan Mahabhiyan (PM-KUSUM) scheme was launched in 2019. This initiative aims to reduce farmers’ dependence on diesel and conventional grid electricity by promoting the use of solar-powered irrigation pumps69. The scheme not only provides financial incentives for the installation of standalone solar pumps but also supports the development of grid-connected renewable power plants, thereby enhancing energy security in rural areas.
In a bid to encourage domestic manufacturing and reduce reliance on imports, particularly from China, the government mandated in December 2024 that, starting June 2026, all clean energy projects must utilize locally produced solar photovoltaic modules. This policy is expected to stimulate local manufacturing, addressing supply chain vulnerabilities and fostering self-reliance in the solar sector70.
Collectively, these policies have created a conducive environment for the development and diffusion of solar PV technology in India, aligning with the nation’s commitment to sustainable energy and climate change mitigation.
- United States
The development and diffusion of Solar Photovoltaic (PV) technology in the United States have been significantly influenced by several key policies. The Energy Policy Act of 2005 was a crucial milestone, providing tax incentives, including the Investment Tax Credit (ITC), which offers a 30% federal tax credit for solar systems installed on residential, commercial, and industrial properties, driving market growth71.The American Recovery and Reinvestment Act (ARRA) of 2009 further accelerated solar energy deployment by allocating significant funds to solar technology development and offering grants for solar installations, stimulating both demand and innovation in the solar industry72.State-level policies, particularly in California, have also been vital. The California Solar Initiative (CSI) and Net Metering policies have provided strong incentives for both residential and commercial solar installations, enhancing solar energy adoption73.
These policies have collectively fostered a robust environment for solar energy growth in the U.S., lowering costs, encouraging innovation.
Positive and Negative Aspects of Alternative Policies: The implementation of alternative policies in the solar photovoltaic (PV) industry has led to both positive and negative outcomes, each playing a crucial role in shaping the growth of the sector. One of the key benefits of these policies is the promotion of market efficiency. Auction-based systems, such as those introduced in Germany’s Renewable Energy Sources Act (EEG), encourage competitive bidding for solar energy contracts, ensuring that energy is procured at the lowest cost. This fosters a more cost-efficient and integrated renewable energy market, helping to streamline the transition to clean energy74. Similarly, financial incentives such as feed-in tariffs (FiTs) in Germany and the U.S. Investment Tax Credit (ITC) are crucial in encouraging technological advancements in solar technology71. These policies offer long-term financial stability to producers, driving innovation and making solar energy more affordable and widespread.
Moreover, subsidies and grants like China’s “Golden Sun” program have been instrumental in rapidly expanding solar PV installations. By offering financial support, China has successfully reduced barriers to entry for local manufacturers, thereby stimulating industry growth75. Additionally, policies that mandate the use of locally produced solar modules, such as India’s National Solar Mission, have not only helped create jobs but also fostered economic development within the renewable energy sector. These measures ensure that the country is building a self-sustaining solar industry. Furthermore, consumer empowerment is a significant advantage in regions like California, where policies such as net metering allow consumers to sell excess energy back to the grid. This encourages greater solar adoption and contributes to the overall energy transition.
However, while these policies have fostered considerable growth, they also bring certain challenges. The over-reliance on subsidies, such as Germany’s feed-in tariffs, can lead to market distortion, potentially resulting in inefficiencies. These subsidies may place a financial burden on governments and can contribute to concerns over solar over-supply and negative pricing76. Auction systems, while promoting efficiency, also introduce uncertainty for investors. Low winning bids can lead to unsustainable prices, making it difficult for smaller projects to compete and potentially stalling the growth of the solar sector.
These alternative policies have both positive and negative implications for the solar PV industry, each contributing to different aspects of the energy transition. Proper management of these policies is essential for achieving sustainable and equitable growth.
References
1 Zhang, C., Zhang, J., Ma, X. & Feng, Q. Semiconductor photovoltaic cells. (Springer, 2021).
2 Cook, G. Photovoltaic fundamentals. (Solar Energy Research Institute, 1991).
3 Reuters. (2025).
4 Feeds, S. Concentrated Solar Power (CSP) Vs Photovoltaic (PV): An In-depth Comparison, <https://www.solarfeeds.com/mag/csp-and-pv-differences-comparison/> (2023).
5 Energy, U. S. D. o. Solar Systems Integration Basics, <https://www.energy.gov/eere/solar/solar-systems-integration-basics> (2025).
6 Marques Lameirinhas, R. A., Torres, J. P. N. & de Melo Cunha, J. P. A photovoltaic technology review: History, fundamentals and applications. Energies 15, 1823 (2022).
7 Richardson, L. Solar history: Timeline & invention of solar panels, <https://www.energysage.com/about-clean-energy/solar/the-history-and-invention-of-solar-panel-technology/> (2023).
8 Society, A.-A. P. April 25, 1954: Bell Labs Demonstrates the First Practical Silicon Solar Cell, <https://www.aps.org/apsnews/2009/04/bell-labs-silicon-solar-cell> (2009).
9 Planet, O. Vanguard 1, the first Solar-Powered Satellite [and the Oldest Human-Made Object Still in Orbit] was Launched on March 17, 1958, <https://ourplnt.com/vanguard-1-first-solar-powered-satellite/> (2023).
10 Powerlutions. The History of Solar Energy in the United States, <https://powerlutions.com/blog/the-history-of-solar-energy-in-the-united-states/> (2023).
11 Delaware, U. o. Over the Years, <https://iec.udel.edu/about/> (2025).
12 Energy, N. E.-C. Evolution of Solar Cost, <https://newenglandcleanenergy.com/panels/evolution-of-solar-efficiency/> (2025).
13 Data, O. W. Solar panel prices have fallen by around 20% every time global capacity doubled, <https://ourworldindata.org/data-insights/solar-panel-prices-have-fallen-by-around-20-every-time-global-capacity-doubled> (2024).
14 Wikipedia. Growth of photovoltaics, <https://en.wikipedia.org/wiki/Growth_of_photovoltaics> (2025).
15 TheFaunaVerse. The Significance of Large Scale Solar Farms in Energy Sustainability, <https://thefaunaverse.com/articles/large-scale-solar-farms-sustainable-energy/> (2025).
16 NREL. Documenting a Decade of Cost Declines for PV Systems, <https://www.nrel.gov/news/program/2021/documenting-a-decade-of-cost-declines-for-pv-systems.html> (2021).
17 Dada, M. & Popoola, P. Recent advances in solar photovoltaic materials and systems for energy storage applications: a review. Beni-Suef University Journal of Basic and Applied Sciences 12, 1-15 (2023).
18 Asmelash, E. & Prakash, G. Future of Solar Photovoltaic: Deployment, investment, technology, grid integration and socio-economic aspects. International Renewable Energy Agency, Abu Dhabi, 1-73 (2019).
19 Weckend, S., Wade, A. & Heath, G. A. End of life management: solar photovoltaic panels. (National Renewable Energy Laboratory (NREL), Golden, CO (United States), 2016).
20 Iea. Evolution of solar PV module cost by data source, 1970-2020, <https://www.iea.org/data-and-statistics/charts/evolution-of-solar-pv-module-cost-by-data-source-1970-2020> (2020).
21 Kumar, R. R., Samykano, M., Pandey, A., Kadirgama, K. & Tyagi, V. Phase change materials and nano-enhanced phase change materials for thermal energy storage in photovoltaic thermal systems: A futuristic approach and its technical challenges. Renewable and Sustainable Energy Reviews 133, 110341 (2020).
22 La Camera, F. Renewable power generation costs in 2020–irena. International Renewable Energy Agency: Masdar City, Abu Dhabi (2020).
23 Vimmerstedt, L. et al. Annual technology baseline: The 2022 electricity update. (National Renewable Energy Lab.(NREL), Golden, CO (United States), 2022).
24 Moore, J., Bullard, N. & Officer, C. C. BNEF executive Factbook. (2021).
25 Bahar, H. Renewables 2020—Analysis and Forecast to 2025. International Energy Agency: Paris, France (2020).
26 Renewables, I. Analysis and Forecast to 2026. International Energy Agency: Paris, France (2021).
27 Administration, U. S. E. I. Solar Energy and the Environment <https://www.eia.gov/energyexplained/solar/solar-energy-and-the-environment.php?> (2024).
28 Scientists, U. o. C. Environmental Impacts of Solar Power, <https://www.ucsusa.org/resources/environmental-impacts-solar-power> (2013).
29 Wikipedia. Solar Power <https://en.wikipedia.org/wiki/Solar_power?> (2025).
30 Wikipedia. Life-cycle greenhouse gas emissions of energy sources, <https://en.wikipedia.org/wiki/Life-cycle_greenhouse_gas_emissions_of_energy_sources?> (2024).
31 Fthenakis, V. M., Kim, H. C. & Alsema, E. Emissions from photovoltaic life cycles. Environmental science & technology 42, 2168-2174 (2008).
32 Stages, L. C. Life cycle greenhouse gas emissions from solar photovoltaics. J. Ind. Ecol 16, S122 (2012).
33 Payback, C. Energy and Carbon Payback Times for
Modern U.S. Utility Photovoltaic Systems (2024).
34 AXIOS. The technology paving the way for the future of solar energy, <https://www.axios.com/sponsored/the-technology-paving-the-way-for-the-future-of-solar-energy> (2024).
35 Delfos. Solar Panel Recycling: The Current State and Future Prospects of Sustainable Energy Solutions, <https://www.delfos.energy/blog-posts/solar-panel-recycling?> (2025).
36 Guardian, T. Australia’s solar waste problem – identified as a priority by the federal government in 2016 – is becoming larger and more urgen, <https://www.theguardian.com/environment/2024/oct/23/australia-renewable-energy-solar-waste?> (2024).
37 Weckend, S., Wade,Andreas Heath,Garvin A. End of life management:solar photovoltic panles. (National Renewable Energy Laboratory (NREL),Golden,CO(United States), 2016).
38 Energy, U. S. D. o. Agrivoltaics: Solar and Agriculture Co-Location, <https://www.energy.gov/eere/solar/agrivoltaics-solar-and-agriculture-co-location?> (2025).
39 How to Address Two Environmental Crises at Once <https://www.newyorker.com/culture/the-lede/how-to-address-two-environmental-crises-at-once?> (2025).
40 Association, S.-S. E. I. Land Use & Solar Development, <https://seia.org/initiatives/land-use-solar-development/?> (2025).
41 Ud Din, S. et al. Assessing public perception and willingness to pay for renewable energy in Pakistan through the theory of planned behavior. Frontiers in Energy Research 11, 1088297 (2023).
42 Baranzini, A., Carattini, S. & Péclat, M. What drives social contagion in the adoption of solar photovoltaic technology. (Grantham Research Institute on Climate Change and the Environment, 2017).
43 Wikipedia. Renewable energy in Germany, <https://en.wikipedia.org/wiki/Renewable_energy_in_Germany?> (2025).
44 Wikipedia. Solar power in California, <https://en.wikipedia.org/wiki/Solar_power_in_California?> (2024).
45 Guardian, T. Balcony solar panels can save 30% on a typical household’s electricity bill and, with vertical surface area in cities larger than roof space, the appeal is clear, <https://www.theguardian.com/environment/2024/dec/18/if-a-million-germans-have-them-there-must-be-something-in-it-how-balcony-solar-is-taking-off?> (2025).
46 Schulte, E., Scheller, F., Sloot, D. & Bruckner, T. A meta-analysis of residential PV adoption: the important role of perceived benefits, intentions and antecedents in solar energy acceptance. Energy Research & Social Science 84, 102339 (2022).
47 Agency, I. R. E. Renewable Energy and Jobs-Annual Review 2021, <https://www.irena.org/publications/2021/Oct/Renewable-Energy-and-Jobs-Annual-Review-2021?> (2021).
48 Group, W. B. Off-Grid Solar Market Trends Report 2020, <https://www.worldbank.org/en/topic/energy/publication/off-grid-solar-market-trends-report-2020?> (2020).
49 Agency, I. R. E. Renewable Energy and Jobs-Annual Review 2022. (2022).
50 Organization, W. H. Air Pollution, <https://www.who.int/health-topics/air-pollution?> (2025).
51 CHAN, H. T. H. Fossil fuel air pollution responsible for 1 in 5 deaths worldwide, <https://hsph.harvard.edu/climate-health-c-change/news/fossil-fuel-air-pollution-responsible-for-1-in-5-deaths-worldwide/?> (2021).
52 Program, U. E. Seven things you should know about household air pollution, <https://www.unep.org/news-and-stories/story/seven-things-you-should-know-about-household-air-pollution?> (2021).
53 Alliance, G.-T. V. In a Nigerian border town, a solar vaccine fridge is changing lives, <https://www.gavi.org/vaccineswork/nigerian-border-town-solar-vaccine-fridge-changing-lives?> (2021).
54 IEA, P. World Energy Outlook 2022. Paris, France: International Energy Agency (IEA) (2022).
55 Agency, I. E. Access to Electricity <https://www.iea.org/reports/sdg7-data-and-projections/access-to-electricity> (2021).
56 college, b. It starts with the sun, <https://www.barefootcollege.org/solution/solar/> (2025).
57 transect. AI solar sentiment: The Social Impacts of Solar Energy, <https://www.transect.com/blog/ai-solar-sentiment> (2023).
58 German Renewable Energy Sources Act, <https://en.wikipedia.org/wiki/German_Renewable_Energy_Sources_Act?> (2024).
59 Feed-in tariffs in Germany, <https://en.wikipedia.org/wiki/Feed-in_tariffs_in_Germany?> (2024).
60 Reuters. Germany to mandate open market sales for new wind, solar plants, <https://www.reuters.com/business/energy/germany-mandate-open-market-sales-new-wind-solar-plants-2024-11-13/?> (2024).
61 DLA PIPER. The zero tax rate on the supply of photovoltaic systems – further clarifications in the newly published BMF circular, <https://www.dlapiper.com/en/insights/publications/2023/12/the-zero-tax-rate-on-the-supply-of-photovoltaic-systems?> (2023).
62 ISE, F. I. f. S. E. S. German Net Power Generation in 2024: Electricity Mix Cleaner than Ever, <https://www.ise.fraunhofer.de/en/press-media/press-releases/2025/public-electricity-generation-2024-renewable-energies-cover-more-than-60-percent-of-german-electricity-consumption-for-the-first-time.html?> (2025).
63 Solarenergie in China, <https://de.wikipedia.org/wiki/Solarenergie_in_China?> (2025).
64 Xiong Yongqing , Y. X. Government subsidies for the Chinese photovoltaic industry. ElSEVIER 99 (2016).
65 Song, X., Huang, Y., Zhang, Y., Zhang, W. & Ge, Z. An Appraisal on China’s Feed-In Tariff Policies for PV and Wind Power: Implementation Effects and Optimization. Sustainability 15, 5137 (2023).
66 Press, A.-T. A. China built out record amount of wind and solar power in 2024, <https://apnews.com/article/wind-solar-energy-china-climate-carbon-emissions-b337503abfacfd9b7829fd7bbcd507e9?> (2025).
67 Reuters. China to roll back clean power subsidies after boom, <https://www.reuters.com/business/energy/china-roll-back-clean-power-subsidies-after-boom-2025-02-09/?> (2025).
68 Energy, M. o. N. a. R. India surging ahead in the field of Green Energy – 100 GW Solar Scale-Up plan, <https://pib.gov.in/newsite/PrintRelease.aspx?> (2015).
69 Energy, M. o. N. a. R. A New Revolution, <https://mnre.gov.in/en/pradhan-mantri-kisan-urja-suraksha-evam-utthaan-mahabhiyaan-pm-kusum/> (2025).
70 R, S. N. India mandates use of locally made solar cells in government projects from June 2026, <https://www.reuters.com/business/energy/india-mandates-use-locally-made-solar-cells-clean-energy-projects-june-2026-2024-12-10/?> (2024).
71 Star, E. Tax Credit Legislation, <https://www.energystar.gov/about/federal-tax-credits/tax-credit-information> (2022).
72 Energy, U. S. D. o. Recovery Act, <https://www.energy.gov/recovery-act> (2021).
73 Comission, C. P. U. California Solar Initiative (CSI), <https://www.cpuc.ca.gov/industries-and-topics/electrical-energy/demand-side-management/california-solar-initiative> (2025).
74 Action, F. M. f. E. A. a. C. Renewable Energy, <https://www.bmwk.de/Redaktion/EN/Dossier/renewable-energy.html> (2025).
75 Yong-hua Wang, G.-l. L. a. H. K. (2017).
76 Comission, E. EU energy policy, <https://energy.ec.europa.eu/index_en> (2024).